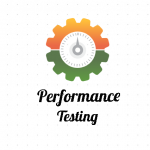
Introduction
The ability to impact a specific sport or activity depends on our capacity for that specific sport or physical activity. Certain sports and activities require the individual to have certain physiological abilities at a certain level. That is becoming a common knowledge with the growth of formal research papers and studies worldwide.
With that said, there is a huge way to cover between being a recreational athlete, professional or elite athlete, with the difference laying in the process; being that someone systematically measures (testing) its own capacity and athletic variables, planning on how to improve them and finally working on and improving them in order to achieve a specific goal. The same rule applies within a clinical setting, for instance heart or lung pathologies and the need of testing to measure the state of function. Without a start (testing), it may be quite challenging to reach any goal.
What is Exercise Physiology?
Exercise physiology is a scientific discipline that focuses on how an organism responds to exercise [1]. Therefore, exercise has been an outstanding model in order to study human and animal physiology and its response to exercise. Most people are familiar with the study of exercise physiology as it relates to sport performance. However, in the last decades it has become apparent that the study of exercise physiology is also relevant in clinical settings. This due to our understanding of how exercise can be used in both the treatment and prevention of multiple diseases including cardiovascular diseases, pulmonary diseases, diabetes, and several types of cancers [1] [2]. The following video describes what exercise physiology is and how that helps performance coaches, physiotherapists and other health specialist applying those principles on the field.
Physiological variables that affect athletic performance?
Multiple physiological variables affect athletic performance, and usually those are separated in terms of testing based on endurance performance and strength/power performance [1]. Among those variables that influence endurance performance, the following are often used and thus will be described further here; percent body fat, VO2max, the anaerobic threshold, anaerobic capacity and running economy [1] [3]–[5]. Several of these variables can differentiate between highly trained and untrained subjects. Nevertheless, within well-trained athletes there is high variability for each. Simply said, highly trained athletes tend to have higher VO2max values than untrained individuals. These high VO2max values are partly responsible for the better endurance performance of the highly trained runners. However, within a group of elite athletes this does not apply the same way, as a person with the highest VO2max values is not necessarily the best runner, nor vice versa. Several examples in the literature have registered elite athletes with moderate VO2max or relatively untrained individuals with quite high VO2max values (likely genetically predisposed).
- 1. %BF - Percent Body Fat
- 2. VO2max – Maximal Oxygen Consumption (Aerobic capacity)
- 3. HRmax – Maximal Heart Rate
- 3.1 Heart Rate Variabiity (HRV)
- 4. LA –Blood Lactate & Anaerobic Threshold (AT)
- 5. Running Economy & Mechanical Efficiency
- 6. Omegawave
It should be noted that results from such measurement should be compared with the population the individual tested belongs to. Average percent body fat values for 20-29 year-old subjects in the general population are 15.9% for men and 22.1% for women. However, the percent body fat among athletes of the majority (not all) of sports are typically lower than those registered in an average population and in few cases as low as that they could have been clinically significant for the average population; Olympic competitor Apolo Ohno 2.8%, elite runners ranging between 4-10% in men and 7-15% in women, and among Olympic wrestler 6% to 12.8% to as low as 2.4% reported [10].
Oxygen consumption is the amount of oxygen entering and utilized by the body [1]. The oxygen entering the body at the level of the lungs is used for the production of ATP in the mitochondria of our cells. Due to the fact that most of the energy in the body is produced aerobically, VO2 can be used to measure the energy a subject is expending. VO2 can be measured in absolute values (L/min) or relative to body mass (ml/kg*min). The latter one being able to compare for instance between to rowers or wrestler with different weight class.
Oxygen consumption depends on i) the ability of the heart to pump out blood, ii) the ability of the tissues to extract oxygen from the blood, iii) the ability to ventilate and iv) the ability of the alveoli to extract oxygen from the air.
Resting absolute values are seen to be around .2-.5L/min among men and .15-.4L/min among women. The approximate resting relative VO2 for all individuals is 3.5ml/kg*min.
Maximal oxygen consumption is the highest VO2 value measured during maximal exercise. VO2max tests can also be used clinically to assess the type and severity of cardiovascular or pulmonary limits to exercise. Certain objective criteria can be used after testing to evaluate on whether or not the peak VO2 value during the test is truly a maximal value. VO2max is used as the best indicator of aerobic capacity and therefore of aerobic performance.
In terms of exercise, VO2max can increase with training. In average an untrained individual can increase his VO2max by approximately 15-20%. However, in well trained athletes increases in VO2max may not be as great, as they may already be nearly as high as they can go. Fortunately for such well-trained athletes, continued training can improve efficiency, such that they can go faster for a given oxygen consumption. Additionally, the percent of VO2max that the athlete can sustain for prolonged periods of time is also very trainable [1]. Please have a look at the following testing procedure for visual illustration REF.
During a VO2max testing in the lab, several other important factors are also analysed. Those factors are:
- b) Carbon Dioxide production VCO2: evaluates buffering of blood and maintenance of pH
- c) Respiratory Exchange Ratio RER: evaluates types of fuel used during exercise
- d) Pulmonary Ventilation VE: evaluates amount of air moved in/out of lungs based on the depth of breathing
- e) Fraction of expired air that is oxygen/carbon dioxide FEO2 (O2/CO2%): evaluates how well O2 is used during gas exchange in alveoli and ration of CO2 produced in the process
- g) Ventilatory equivalent ratio for oxygen and carbon dioxide VE/VO2 & VE/VCO2: Evaluates ventilation increases and blow-off excess of CO2, sth important during increase of lactic acid in order to prevent blood from being too acidic.
First thing tested related to HR is the cardiac rhyrhm, with a HF lower than 60bpm defined as bradycardia while HF higher than 100bpm defined as tachycardia. Other heart diseases should be taken into consideration as they have an impact on combined tests such as VO2max test. An individual with heart failure have low stroke volumes which reduces the cardiac output and therefore results in a lower VO2max.
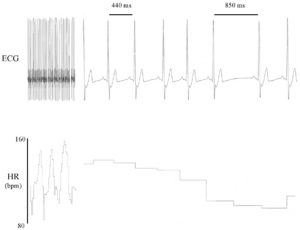
Picture taken by George Billman
And as Simon Wegerif mentioned in one of his interviews “the vast majority of endurance athletes don’t appreciate the simple truth that you don’t get fitter during exercise; instead you get fitter recovering from exercise.”
Lactic acid (LA) is one of the products of anaerobic carbohydrate metabolism in the cells. It was earlier believed to be a waste product, but later research has shown its importance to anaerobic work.
The amount of lactic acid in the blood is dependent on both how much it is produced and how much that is eliminated (produced and cleared from the circulation). This reflects i) the intensity of the exercise for the subject (did the subject give a maximal effort), ii) the degree to which the subject needed to supplement aerobic energy production with anaerobic energy production and iii) the subject’s lactic acid tolerance.
One reason why blood lactate is an important variable to assess is that the hydrogen ions that result from lactate production in the cell are known to cause muscle fatigue via several different mechanisms [11]–[13]. On the other hand, during high intensity exercise, the ability to use anaerobic energy systems is very important [14]. Thus, it is not uncommon to find very high maximal blood lactate values in athletes competing in high intensity events.
The anaerobic threshold (AT) is an important predictor of endurance performance. At intensities lower than the anaerobic threshold, almost all of the energy needed during the exercise is coming from aerobic energy systems. On the other hand, at intensities above threshold, anaerobic energy production is needed to supplement aerobic energy production. Take in mind that there is not something “either or”, those systems complement each other and research has shown that even during maximal efforts, there is aerobic contribution between 1-2min [15].
The standard process of assessing the AT is by testing blood lactate (LA) continuously throughout the test and identifying the point where blood LA starts to accumulate, something also known as “the lactate threshold” [1]. Although a simple method for exercise physiologist, there are few times that it is not always convenient to take blood lactates during the test. Luckily, there is a non-invasive means of mapping the AT using the ventilatory response to exercise. There are several methods applied that ventilatory threshold can be determined[1], with the VE/VO2 point where it begins to continuously increase being the one often used [16].
Resting blood lactate for a healthy individual is around 1 mM, while the anaerobic threshold is detected around 4 mM. I mentioned earlier that there are certain criteria to be met for a valid VO2max test, with a value exceeding 8 mM being one of them. Click here to see the blood lactate (LA) response to exercise.
Physiological testing
Physiological testing attempts therefore to analyse the factors mentioned above and evaluate i) their level, ii) their significance based on your sport/activity and iii) the potential of improvement. The following testing procedures are recommended to be performed by exercise physiologist, clinical physiologist and physiotherapist working with (clinical) testing.
- Vo2 Max Testing (with lactate threshold testing if desired)
- Lactate testing (threshold and at different zones)
- Combined VO2max & Lactate Threshold Test
- Spirometry Testing (Lung Function)
- (Keiser) Strength Training and Testing
- Body Composition Testing
- Sport-specific Field Testing
- Blood Testing
How can physiological testing help athletes and coaches/physiotherapists?
Before summarizing and coming to a conclusion, there is one conclusion to make and one thing to be sure about. No laboratory test alone can predict with 100% accuracy how an athlete will perform in the field, although several laboratory tests correlate well with actual performance measures.
Despite the fact that assessing an athlete’s performance in the field could provide a better assessment of performance abilities, isolating individual physiological parameters that influence performance and evaluate them is quite challenging or even impossible this way. Therefore, one major benefit of physiological testing in laboratories is that they allow us to better isolate specific elements related to performance.
For untrained individuals that would be mapping gaps of training variables and improving them based on type of training needed for that particular variable. Within trained individuals within a sport would be for instance to map which energy systems each one of them use to perform a certain task. An example of personal testing of two young athletes at the same age competing in cross country skiing 10km. One having a great aerobic capacity while the other great anaerobic, putting them at a quite close level of performance. Another example between to other athletes having A: superior VO2 max with inferior utilization while B: inferior VO2 max with superior utilization.
Therefore, improvements in these athletes may require different training strategies.
Benefit of appropriate physiological testing and test interpretation can:
- Isolate specific physiological variables that influence performance
- Identify an athlete’s physiological strengths and weaknesses
- Repeat testing allows athletes and coaches to monitor the effectiveness of training
- Allows athletes and coaches to better individualize training
- May provide insight into the athlete’s or individuals health status such as:
- Disease
- Injury
- Overtraining
- Fatigue
Physiological testing should be used as a tool. It cannot predict gold medal performances and it should be noted that exercise physiology is only one piece of the exercise performance tool-box. Exercise performance at the highest levels of competition are also dependent upon:
- Psychology and motivation
- Genetics
- Biomechanics
- Health (and injuries)
References
- V. L. Katch, W. D. McArde, and F. I. Katch, Essentials of Exercise Physiology, no. 1. 2011
- R. Ramsey and K. Strohl, “Peter G. Gibson (ed): Evidence-based respiratory medicine,” Sleep Breath., vol. 10, no. 4, pp. 217–218, Nov. 2006.
- J. D. MacDougall, H. A. Wenger, and H. J. Green, “Physiological Testing of the High-Performance Athlete.,” Med. Sci. Sport. Exerc., vol. 25, no. 2, p. 305, 1993.
- P. Ford, M. De Ste Croix, R. R. Lloyd, R. Meyers, M. Moosavi, J. Oliver, K. Till, C. C. Williams, and M. D. S. Croix, “The long-term athlete development model: physiological evidence and application.,” J. Sports Sci., vol. 29, no. 4, pp. 389–402, 2011.
- G. D. Wells and S. R. Norris, “Assessment of physiological capacities of elite athletes & respiratory limitations to exercise performance,” Paediatric Respiratory Reviews, vol. 10, no. 3. pp. 91–98, 2009.
- A. B. Loucks, “Energy balance and body composition in sports and exercise.,” J. Sports Sci., vol. 22, no. 1, pp. 1–14, 2004
- J. R. Moon, “Body composition in athletes and sports nutrition: an examination of the bioimpedance analysis technique.,” Eur. J. Clin. Nutr., vol. 67 Suppl 1, no. S1, pp. S54–9, 2013.
- R. M. Malina, “Body Composition in Athletes: Assessment and Estimated Fatness,” Clinics in Sports Medicine, vol. 26, no. 1. pp. 37–68, 2007
- J. Parizkova, V. Bunc, S. Sprynarova, E. Mackova, and J. Heller, “Body composition, aerobic capacity, ventilatory threshold, and food intake in different sports.,” Ann. Sport. Med., vol. 3, no. 3, pp. 171–177, 1987.
- R. Kordi, R. Nourian, M. Rostami, and W. A. Wallace, “Percentage of body fat and weight gain in participants in the tehran high school wrestling championship.,” Asian J. Sports Med., vol. 3, no. 2, pp. 119–25, Jun. 2012.
- R. Kordi, R. Nourian, M. Rostami, and W. A. Wallace, “Percentage of body fat and weight gain in participants in the tehran high school wrestling championship.,” Asian J. Sports Med., vol. 3, no. 2, pp. 119–25, Jun. 2012.
- F. S. Routledge, T. S. Campbell, J. A. McFetridge-Durdle, and S. L. Bacon, “Improvements in heart rate variability with exercise therapy.,” Can. J. Cardiol., vol. 26, no. 6, pp. 303–12, 2010.
- C. Schubert, M. Lambertz, R. A. Nelesen, W. Bardwell, J.-B. Choi, and J. E. Dimsdale, “Effects of stress on heart rate complexity—A comparison between short-term and chronic stress,” Biol. Psychol., vol. 80, no. 3, pp. 325–332, 2009.
- L. Salahuddin, J. Cho, M. G. Jeong, and D. Kim, “Ultra short term analysis of heart rate variability for monitoring mental stress in mobile settings.,” Conf. Proc. … Annu. Int. Conf. IEEE Eng. Med. Biol. Soc. IEEE Eng. Med. Biol. Soc. Annu. Conf., vol. 2007, pp. 4656–9, 2007.
- H. M. Seong, J. S. Lee, T. M. Shin, W. S. Kim, Y. R. Yoon, and Y. R. Yoon, “The analysis of mental stress using time-frequency distribution of heart rate variability signal.,” Conf. Proc. … Annu. Int. Conf. IEEE Eng. Med. Biol. Soc. IEEE Eng. Med. Biol. Soc. Annu. Conf., vol. 1, pp. 283–5, 2004.
- P. K. Stein, P. Nelson, J. N. Rottman, D. Howard, S. M. Ward, R. E. Kleiger, and R. M. Senior, “Heart Rate Variability Reflects Severity of COPD in PiZ α1-Antitrypsin Deficiency,” Chest, vol. 113, no. 2, pp. 327–333, 1998.
- J. M. Dekker, R. S. Crow, A. R. Folsom, P. J. Hannan, D. Liao, C. A. Swenne, and E. G. Schouten, “Low heart rate variability in a 2-minute rhythm strip predicts risk of coronary heart disease and mortality from several causes: the ARIC Study. Atherosclerosis Risk In Communities.,” Circulation, vol. 102, no. 11, pp. 1239–44, Sep. 2000.
- S. H. VANDER A, Sherman J, Luciano D, Widmaier E, Raff H, Human Physiology: The Mechanism of Body Function, 8th Edition, vol. 40. 2001.
- D. G. Allen, G. D. Lamb, and H. Westerblad, “Skeletal muscle fatigue: cellular mechanisms.,” Physiol. Rev., vol. 88, no. 1, pp. 287–332, 2008.
- H. Ishii and Y. Nishida, “Effect of Lactate Accumulation during Exercise-induced Muscle Fatigue on the Sensorimotor Cortex.,” J. Phys. Ther. Sci., vol. 25, no. 12, pp. 1637–42, 2013.
- G. Van Hall, “Lactate as a fuel for mitochondrial respiration,” in Acta Physiologica Scandinavica, 2000, vol. 168, no. 4, pp. 643–656.
- P. B. Gastin, “Energy system interaction and relative contribution during maximal exercise.,” Sports Med., vol. 31, no. 10, pp. 725–41, 2001.
- S. E. Gaskill, B. C. Ruby, a J. Walker, O. a Sanchez, R. C. Serfass, and a S. Leon, “Validity and reliability of combining three methods to determine ventilatory threshold.,” Med. Sci. Sports Exerc., vol. 33, no. 11, pp. 1841–1848, 2001.
- A. Santos-Lozano, A. M. Angulo, P. S. Collado, F. Sanchis-Gomar, H. Pareja-Galeano, C. Fiuza-Luces, A. Lucia, and N. Garatachea, “Aging’s effects on marathon performance insights from the New York City race.,” Int. J. Sports Physiol. Perform., vol. 10, no. 7, pp. 840–7, Oct. 2015.
- B. Lara, J. J. Salinero, and J. Del Coso, “The relationship between age and running time in elite marathoners is U-shaped.,” Age (Dordr)., vol. 36, no. 2, pp. 1003–8, Apr. 2014.